Cancer treatment is evolving rapidly. New techniques can identify and target tumor vulnerabilities while leaving healthy cells alone. Immunotherapy can harness the body’s immune system to fight cancer. But new treatments still don’t work for everyone. We need more breakthroughs.
To find out what’s in the pipeline, we turned to the Damon Runyon Cancer Research Foundation. This organization provides multiyear funding for scientists, often at the beginning of their careers, who have “bold and brave” ideas and connects them with a worldwide network of mentors. The result is a long string of cancer firsts—and 12 Nobel prizes. So we asked Damon Runyon to help us identify five researchers they have supported who are doing transformative work. Welcome to the future of cancer care.
Jeremy Copperman, PhD: Harnessing Big Data
When Jeremy Copperman, PhD, defended his thesis in statistical physics, he was finishing treatment for Hodgkin lymphoma. After his recovery, his priorities changed, and he decided he wanted to focus on fighting cancer.
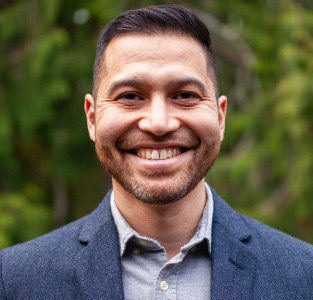
Jeremy Copperman, PhDCourtesy of Jeremy Copperman/Whitney Bradshaw
“My motivations are personal,” he says. “As a survivor, I wanted to see whether the things I’ve worked on in my research career could teach us anything about cancer. I’m a physicist by training trying to learn cancer biology now.”
Working with mentors Daniel Zuckerman, PhD, and Joe Gray, PhD, at Oregon Health and Science University (OHSU), Copperman is studying how machine learning and artificial intelligence can help researchers understand how cancer cells continually adapt to gain the ability to metastasize or develop resistance to treatment—and how to adapt treatment in response.
“There are a lot of new targeted cancer medicines plus a lot of proven existing medicines, but how do you know when to use what and what will be effective at what stage in a cancer that is rapidly changing? Here at the OHSU Knight Cancer Institute, researchers with the SMMART (Serial Measurements of Molecular and Architectural Responses to Therapy) program are almost characterizing the cancer cell population in real time. It’s really changing and adapting treatment on the fly,” Copperman says.
Using repeated tumor biopsies from patients with advanced cancer, a multidisciplinary team analyzes tumor proteins, gene mutations, immune system interactions and cell structure and behavior. Copperman believes the data-driven cell modeling he’s developing can contribute to the personalized management of metastatic disease.
“I’m looking at living cells through a microscope—basically movies of cells—and building models of how they behave,” he explains. “How would that cell migrate through tissue, for instance? If you have a model, you can predict that. And once you can predict it, perhaps you can control it.”
All that analysis yields a massive amount of data, which is where artificial intelligence comes in.
“The beauty of machine learning is that data can have a lot of dimensions, more than a human brain can take in. But the machine can take all these different measurements and learn patterns that a human doctor can’t see,” Copperman says. “It’s a way to pull out predictions and inferences from large amounts of information. You don’t have to know what is important ahead of time—the machine will learn that.”
Now in remission for four and a half years, Copperman is grateful to have the support to move into a new field of research.
“I don’t know if you know many physicists, but the work can be very abstract,” he says. “I want to be able to connect all those dots where I can get from an almost atomic level understanding of what’s happening with the interactions of specific molecules all the way to stopping a cancer cell from becoming metastatic. I want to know that the work I’m doing is going to do some good for a cancer patient.”
Geoffrey Oxnard, MD: A New Kind of Blood Test
While working at Dana-Farber Cancer Institute in Boston, Geoffrey Oxnard, MD, helped develop a blood test that can detect more than 50 types of cancer, often at an early, more treatable stage. But blood tests known as “liquid biopsies” can do much more, revealing whether cancer is progressing and whether a tumor has mutations or other features that make it susceptible to specific targeted therapies or immunotherapies.
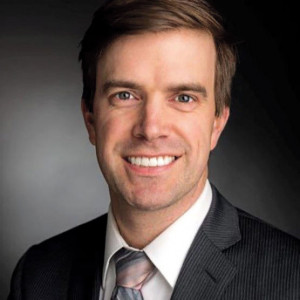
Geoffrey Oxnard, MDCourtesy of Geoffrey Oxnard, MD
“I was a physician and academic researcher focused on lung cancer for almost 10 years, but I found myself thinking about liquid biopsies more and more,” Oxnard says. So last June, he joined Foundation Medicine, which is developing the technology for clinical use.
Liquid biopsies are used for next-generation sequencing (NGS), a process that can characterize hundreds of genes at once instead of laboriously analyzing them one at a time in a tumor tissue sample. NGS is now covered by Medicare and many commercial insurers.
Last fall, the Food and Drug Administration (FDA) approved Foundation Medicine’s FoundationOne Liquid CDx test, which analyzes more than 300 genes, as a companion diagnostic test for eight targeted therapies for breast, ovarian, prostate and lung cancers. Another approved test from Guardant Health, Guardant360 CDx, analyzes 55 genes.
“The dilemma that got us here is a world where cancer patients have a growing number of therapies available and doctors are trying to figure out how to get them the right ones,” Oxnard says. “How do you move away from the historical precedent of chemotherapy for all? That’s precision cancer medicine.”
Testing tumors directly is not always feasible—for example, because enough tumor tissue may not be available. Moreover, ongoing monitoring would require multiple invasive biopsies. Drawing blood is much less invasive, and blood is rich in DNA—some of which comes from cancer cells.
“If you can just find that needle in the haystack, you actually can get an actionable result from a blood test,” Oxnard says. “That’s the promise of the liquid biopsy: to characterize a tumor and figure out its biology—what makes it tick. You send off the blood, and within a week or two, you get back information about the cancer that tells you what to do next.”
More aggressive and metastatic cancers shed more DNA, and changes in the amount of DNA detected over time can be used to gauge disease progression and response to treatment.
“After surgery, you can see if the cancer goes away. The blood test is negative. But if the DNA comes back positive, it tells you the cancer is reemerging,” Oxnard explains. “We can give a treatment and the DNA shedding goes down. If the patient develops resistance, the shedding goes back up. So this dynamic phenomenon creates an opportunity for monitoring. It makes us more nimble in how we care for cancer patients.”
Oxnard’s team is working to develop new uses for liquid biopsies. For example, KRAS mutations are common to many tumors. If they’re detected in the blood, can epigenetics and methylation patterns—chemical tags that turn DNA on or off—reveal whether they came from a pancreatic, colon or lung tumor?
While these possibilities are being explored, liquid biopsies have already “democratized access to tumor profiling,” Oxnard says. “You can get next-generation sequencing of any advanced cancer anywhere in the United States and maybe anywhere in the world. That creates access to this new horizon, tapping into the promise of precision cancer therapy.”
Marcela Maus, MD, PhD: Taking a BiTE Out of Tumors
A new type of immunotherapy, CAR-T, or chimeric antigen receptor T-cell therapy, involves collecting T cells from a patient, adding receptors so the cells can recognize and attack that person’s cancer and reinfusing them back into the body. While CAR-T offers a new option for treating blood cancers, so far it has not proved very effective against solid tumors. But Marcela Maus, MD, PhD, of Harvard Medical School and the Massachusetts General Hospital Cancer Center, hopes to change that.
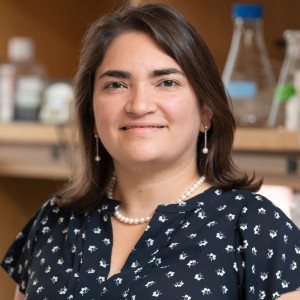
Marcela Maus, MD, PhDCourtesy of Massachusetts General Hospital
“I come from an immunology background,” she says. “The human immune system is so incredibly powerful that we think it can be leveraged against multiple solid tumors.”
Maus and her collaborators are now designing next-generation CAR-T therapies, aiming to create modified T cells that can locate and kill tumor cells while sparing healthy tissue. “Engineering the T cell itself, which is the kind of cell that actually kills cancer, is a powerful strategy because you can add in new genes to redirect it or force it to penetrate cold tumors,” she explains, referring to tumors that are not usually susceptible to immunotherapy.
Maus’s team is working on so-called armored CARs that attack glioblastoma, the deadly brain cancer that killed President Joe Biden’s son Beau. These modified T cells are designed to target a specific mutation that is found only on glioblastoma cells. But the CAR-T cells alone failed to do the trick.
“We gave it to patients with glioblastoma in a trial back in 2015, and we saw that the CAR-T cells were actually able to get into the brain tumor. But because not every single tumor cell has the mutation, the tumors ended up growing back lacking that mutation. We also learned that the body’s immune system would block the CAR-Ts.”
So Maus and her colleagues gave the engineered T cells an extra gene that produces a T-cell engaging antibody-like molecule—more commonly known as a bispecific T-cell engager (BiTE)—that links T cells and their cancer targets.
“A bispecific engager is a synthetic antibody-like molecule that has two business ends,” she explains. “One end targets a tumor molecule, and the other binds to a T cell and activates it. So by forming a little bridge between the T cell and the tumor cell, you can get a T cell to kill it, even though the T cell itself is not specific for the tumor—it will just kill it because it’s so close.”
These molecules are normally too large to enter the brain if injected into the bloodstream, but T cells can cross the blood-brain barrier and deliver them to the tumor.
Maus and her team found that CAR-T cells with bispecific engagers led to complete remission in mice, and they’re now planning to start the first Phase I clinical trial for glioblastoma patients within the next six months.
She thinks it should be possible to use this approach for other brain tumors and perhaps other types of cancer as well. The researchers are also starting to look at ways tumors can evade an attack by T cells, with the aim of developing strategies to get around them.
“Our goal is always to make our work relevant to patients,” she says. “I’m a human doctor, not a mouse doctor. We use mice to get a sense of whether something is going to be effective and safe and to try to pick the strategy that’s most likely to be the winner. But our goal is to make patients’ lives better.”
Jakob Dupont, MD: Off-the-Shelf CAR-T
A major challenge of CAR-T therapy is that it must be custom-made for each patient—a costly and time-consuming process. But what if these cells could be mass-produced and given to multiple people?
“Cell therapy could be a real game changer, and we’re particularly focused on off-the-shelf approaches that can help treat large numbers of patients in a more practical manner,” says Jakob Dupont, MD, the global head of research and development at Atara Biotherapeutics in South San Francisco.
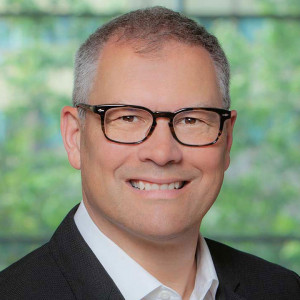
Jakob Dupont, MDCourtesy of Jakob Dupont, MD
While working with his mentor, Richard O’Reilly, MD, at Memorial Sloan Kettering Cancer Center, Dupont was part of a team that developed a CAR-T therapy that targets Epstein-Barr virus (EBV), which can cause certain types of lymphoma and nasopharyngeal cancer.
“What we use are essentially T cells from healthy donors that we educate against Epstein-Barr virus proteins and then generate a population of T cells that are EBV-specific,” he says.
This research started in the late 1990s, before the first commercial custom-made CAR-T therapies were approved. Now Atara is working to bring off-the-shelf T-cell products to market. Not only is the process faster and cheaper than engineering cells for each individual patient, it can also benefit people whose own T cells are damaged.
“You’re not necessarily working with the best T cells, because the patients have received chemotherapy, radiation therapy, and they also have an underlying cancer, so their immune cells are not functioning terribly well,” Dupont explains. “Instead, we’re using normal, healthy T cells that should be more active.”
These EBV-specific T cells can be given to patients who develop lymphoma after an organ transplant because the immunosuppressive drugs they take allow the virus—which most healthy people carry—to multiply and cause disease. The product, dubbed tab-cel, is in Phase III trials, the final step before FDA approval.
“If you give these T cells to these patients, it actually treats—and in many cases cures—this aggressive lymphoma,” Dupont says. “We can also use these off-the-shelf EBV T cells to treat multiple sclerosis, which is thought to be driven by EBV as well.”
What’s more, the EBV-specific T cells can be used as a base to insert genes for additional receptors that recognize tumor antigens, such as CD19, which is found on
B cells that grow out of control in people with leukemia or lymphoma.
“We can also make other CAR-T cells that work against solid tumor antigens, like mesothelin, which we can use to target tumors, including mesothelioma, non-small-cell lung cancer, ovarian cancer or pancreatic cancer,” he says. “So the bottom line is, these off-the-shelf T cells are a great foundation, and we can make many different flavors.”
Atara also has a tool kit of accessory molecules that can be inserted into the CAR-T cells to make them work better and last longer as well as a molecule that stops PD-1, an immune system checkpoint, from turning off T-cell activity. “This is sort of a way to rev up the cancer-fighting potential of those CAR-T cells—we refer to them as armored CARs,” Dupont says. “So you don’t have to give a checkpoint inhibitor drug because it’s essentially already built into the T cells.”
With 20 years of experience as a tumor immunologist, Dupont shifted to industry to help bring therapies to the clinic to improve care for people with cancer.
“I believe a patient’s own immune system holds great promise to treat cancer, and it’s a matter of finding out how to augment the immune system to be as effective as possible,” he says. “But when you can’t necessarily wake up a patient’s own immune system, you can provide a strong immune response from outside. I think that could have a great impact, especially for patients whose immune system is simply not sufficient to fight their cancer on its own.”
Joshua Brody, MD: Vaccines That Fight Cancer
We’re all familiar with vaccines that prevent disease, but similar approaches that train the immune system can also be used to treat cancer.
Joshua Brody, MD, director of the lymphoma immunotherapy program at the Icahn School of Medicine at Mount Sinai in New York City, is working on a promising new “in situ” cancer vaccine that, when injected into a single tumor, activates the immune system to fight cancer throughout the body.
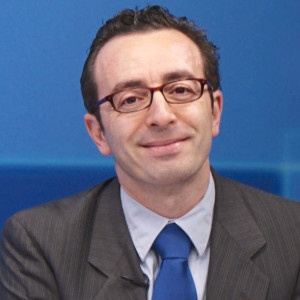
Joshua Brody, MDCourtesy of Joshua Brody, MD
“Therapeutic vaccines—as opposed to prevention vaccines—teach your immune system to fight a problem you already have. In situ means that instead of developing the vaccine in a lab or a factory, we are actually inventing the vaccine at the site of our patient’s tumor—a vaccine made at the site,” Brody explains.
“Every vaccine you’ve ever heard of takes some feature of the thing you want the immune system to recognize and attack, known as an antigen, and puts it alongside something that turns on the immune system,” he continues. “But that’s tough to do with cancer because, while COVID-19 or the flu are the same for everyone, everybody’s cancer is different, so it’s harder to make one standard vaccine.”
Brody’s approach combines a growth factor that stimulates the production of immune dendritic cells, a low dose of radiation and a second drug that triggers an immune response.
“The dendritic cells are the generals of the immune army, which tell all the soldiers—the T cells—what to do. We inject a protein called Flt3L into a tumor, which steps up production of dendritic cells,” Brody explains. “Then we load those dendritic cells with tumor antigens using a mini-dose of radiation. The radiation kills some of the tumor cells, and as they’re dying, their antigens spill out and can be eaten up by the dendritic cells. Then, number three, we activate those dendritic cells with a little stretch of RNA that looks like a virus, even though it’s not a virus. We’re basically putting something into the tumor to teach the immune system to think of it like an infection.”
Preliminary results from a clinical trial that tested the triplet vaccine approach in people with low-grade lymphoma showed that some patients experienced partial or complete remission that sometimes lasted for months or years.
A follow-up trial is testing the vaccine plus a checkpoint inhibitor drug in people with lymphoma, breast cancer or head and neck cancer (NCT03789097).
“We start with tumors that are superficially accessible, meaning you can poke a needle right into them. You could do it for deeper tumors, like pancreatic cancer or lung cancer, but it’s trickier,” Brody says.
“If a person has cancer throughout their body, you only have to teach the immune system to recognize it at one site, and then the immune cells can travel around the whole body to get rid of cancer everywhere.”
Comments
Comments